Filling the Void
Deep Inside UCSB's Quest to Discover the God Particle
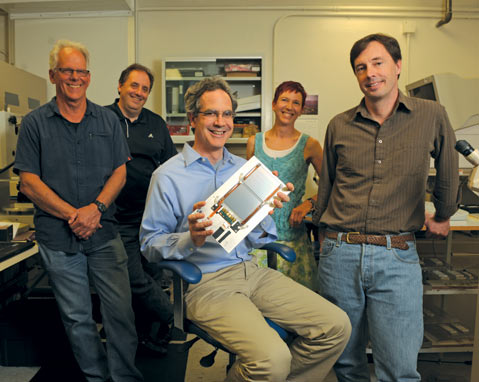
Earlier this month, you may have read about the discovery of something called the Higgs boson, more fabulously known as the “God particle.” You might have also noticed that the man who shared the news with the whole world from a facility in Switzerland is a professor from our very own UC Santa Barbara. But amid the headlines and hoopla, you may have never figured out why the discovery is so important, what UCSB had to do with it, or what the heck a Higgs boson is.
That’s okay. These days, while most of us can’t get through high school without a basic understanding of atomic structure — electrons orbiting around nuclei composed of protons and neutrons — very few of us learn much about the smaller subatomic particles such as quarks, muons, gluons, and bosons. Even if we did, we’d find the mathematical formulas that describe these obscure particles and their relationships with the universe far above our heads. So that is why, as in the case of the recent discovery, physicists must resort to crude metaphors that shed a bit of light on their work when communicating with the rest of us.
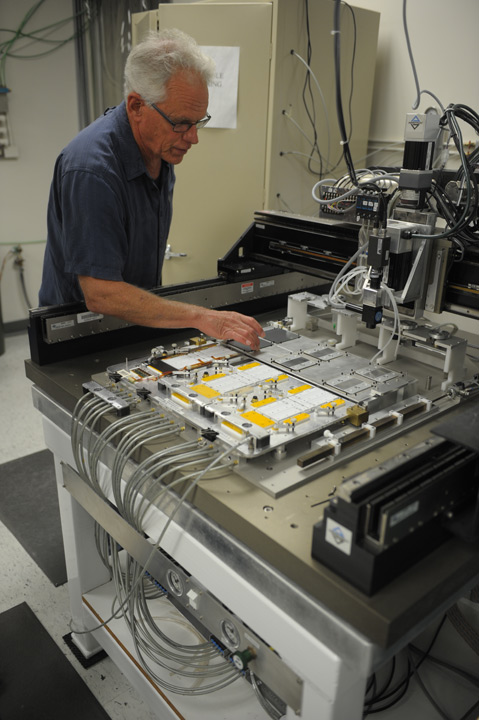
To explain the Higgs boson, physicist David Stuart — a member of UCSB’s High Energy Physics (HEP) group who left Switzerland a day before his campus colleague Joe Incandela broke the big news — opted for “the paparazzi effect,” a popular metaphor being used around the globe to illustrate the biggest discovery in particle physics since at least 1983. “Imagine if President Barack Obama were walking across campus,” explained Stuart two days after the announcement, waving toward the quad outside his fifth-floor office window in Broida Hall. “People would crowd around him and want to talk to him and take his photo. He wouldn’t get too far because his environment would slow him down.” Compare that to a random undergrad walking the same quad, unencumbered by the paparazzi and able to move freely and quickly along.
In that metaphor, the paparazzi are like the Higgs field (the omnipresent fabric of space that was theorized by Scottish physicist Peter Higgs in 1964) and Obama and the undergrad represent particles with differing amounts of mass — the president being massive, the undergrad being nearly massless. Though the theory about what gives objects mass was conceived nearly 50 years ago, the critical component to proving it had yet to be found, leaving a gap in the Standard Model of physics. But this year, the physicists finally located possible members of the paparazzi and were able to show evidence of the existence of the Higgs boson, which would thereby confirm the field and its role in creating mass. “The field permeates the universe,” explained Stuart. “If you excite the field, you will produce the particle.”
Nevertheless, it’s hard to know where to place the discovery of the Higgs boson in the filing cabinet of the human mind. It is at once a momentously important particle, and at the same time, it is invisible and abstract, only detectable by extremely intricate scientific instruments and only able to be created by a 27-kilometer-long particle accelerator that cost $9 billion to build. Combine something too small to see with ideas too big to grasp, and wrap it up in a metaphor that barely works, and it’s easy to see why physicists can come across as high priests nurturing arcane knowledge about the mysteries of the universe. When the physicist Leon Lederman called his 1993 book about the Higgs boson particle The God Particle — he wrote that he actually wanted to call it the “Goddamn particle” because it was so hard to find — it only reinforced that perception.
But most physicists say nothing is further from the truth. As imperceptible as the forces governing our universe are to the naked eye, they are real, they are empirical, and they are material. This is not religion or even metaphysics — it is cold, hard science, and the real magic is in the technology.
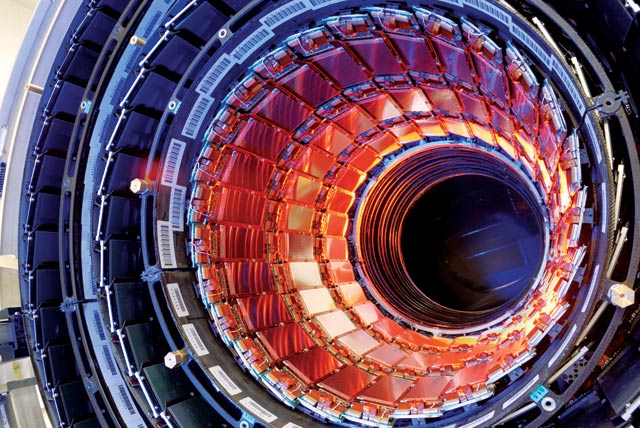
The Collider
The Higgs boson discovery took place a half-world away from UCSB’s Broida Hall in Geneva, Switzerland, at the facility known as CERN, known in English as the European Organization for Nuclear Research. (The “C” is a 60-year-old remnant of when the organization was merely a council.) CERN’s signature piece of equipment is the Large Hadron Collider, or LHC, a 27-kilometer-long (roughly 17-mile-long) underground tunnel that crosses under the French border and qualifies as the largest particle accelerator in the world.
The LHC’s purpose is to crash particles into each other at extremely high speeds so that scientists can then document the resulting smithereens. Think of a Formula One track with two races going on at the same time in opposite directions, but rather than cars, the participants are protons in beams the diameter of a human hair. Each beam contains a hundred thousand million protons (seriously), circling at nearly the speed of light, each making 11,245 revolutions around the 27-kilometer tunnel per second. In 10 hours, they travel the equivalent of the distance to Neptune and back. Every time the two beams pass each other, only about 20 protons in each actually collide, but those collisions add up quickly. And they are all recorded. The LHC produces energies that have not occurred naturally since the Big Bang, and the bigger the collider, the closer to the beginning of time we can get — or, more precisely, the closer we can get to the amount of energy released when our universe originated.
So what’s the point? “The rules of nature that govern how energy is converted into matter are part of what we are trying to figure out,” explained UCSB physicist Jeff Richman, the most senior researcher in the HEP group. In other words, when the grown men and women with supersized brains who staff the LHC are allowed to smash their toys together, we all learn a bit more about how the universe came to be.
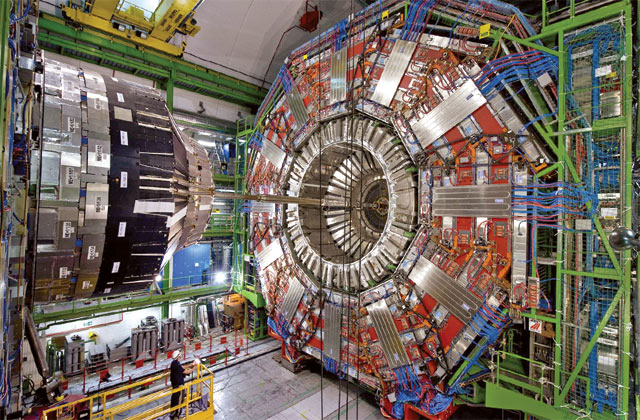
The Detector
The collisions take place right at the center of a detector called the Compact Muon Solenoid (CMS), which, despite its name, is five stories tall. Aided by countless postdoctoral fellows, graduate students, undergrads, and three engineers, four UCSB professors — Richman, Stuart, Incandela, and Claudio Campagnari — played a critical role in both building the instrument and conducting experiments with it.
The CMS is a large cylinder, the cross-section of which, at first glance, looks a bit like the inside of a kaleidoscope. Composed of concentric layers of sensors surrounded by a superconducting magnet and lowered 100 meters into the LHC piece-by-piece from a warehouse above, the CMS tracks exactly what happens to the protons when they collide and decay into new particles. Imagine two volleyballs bumping into each other at East Beach, explained Richman, with two Frisbees flying out of the collision. But then the discs turn into something else, and those turn into something else, and so on. Using electronic imaging similar to that in a camera, the CMS tracks those decay patterns, and then physicists reverse engineer those images into evidence of a Frisbee … er … a boson.
The detectors that measure particle trajectories are deep inside the CMS, where 12,000 overlapping silicon wafers form a barrel-shaped tracking system. Of those wafers, 4,500 were fabricated in a UCSB clean room, where all occupants must wear full bodysuits and booties to keep dust from circulating. Each wafer features 800 channels with microscopic wires and sub-detectors hooked up to microchips. In all, UCSB scientists, technicians, and students bonded more than one million wires to circuits with an instrument that Richman likens to “a giant sewing machine.” This painstakingly intricate work took one-and-a-half years from 2005 to 2006, but the tracking system can now pinpoint the location of a particle to within a thousandth of an inch.
But the work really goes back to 2000, when UCSB — which already had a strong reputation for designing silicon electronics — started the process of designing, building, testing, and calibrating the CMS tracking system. After 12 years, the data is flowing back from Geneva to Broida Hall, where on the fifth floor a couple of weeks ago, two postdocs, Victor Pavlunin and Tom Danielson, were watching numbers dance across their computer screens. Their job is to write software that processes and analyzes the LHC-produced decay patterns, only a tiny fraction of which will offer evidence of the short-lived Higgs boson. Although scientists continue to confirm that the particle they detected is actually the Higgs boson, Pavlunin is already jonesing for the LHC to produce some new particle that will upend, or at least complicate, the Standard Model.
“We are discoverers,” said Pierre Wiltzius, physicist and dean of Mathematical, Life, and Physical Sciences at UCSB. “As soon as we discover something and understand it, it becomes boring. … That is very much the scientist’s mind.”
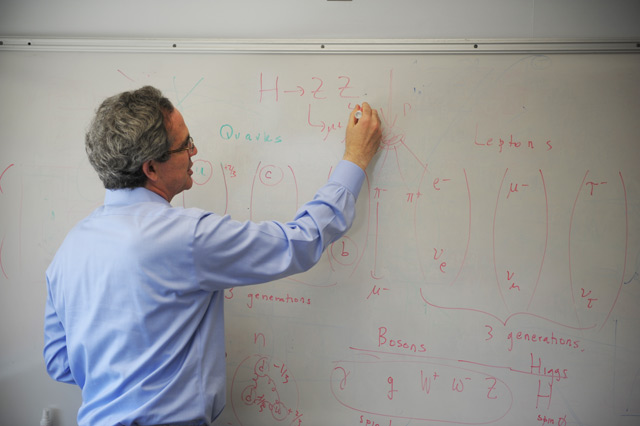
The Upshot
There are certainly practical spin-offs that come from the work done by high-energy physicists — the World Wide Web came out of CERN as a means for scientists to communicate, for instance, and some of the associated imaging technology has greatly benefited the medical world — but their work is much more about understanding the universe than commercial applications. For UCSB, however, being involved in this research comes with a number of tangible perks.
“Getting UCSB to be known [for the Higgs boson discovery] is incredibly important,” said Wiltzius. “It helps us keep growing the atmosphere of excellence here, which helps us keep attracting young, promising faculty. It will help with the retention of faculty who will get offers from very good schools.” The publicity, added Chancellor Henry Yang, “will certainly be most helpful with our fundraising campaign.”
For the physicists involved, the rewards are even more altruistic. “Our product,” said Richman, “is the science and highly trained students and postdocs.” That attention to education has translated to what Wiltzius said is an increasing number of undergrads choosing physics as their major over the past three years, but physics at UCSB has been on an upswing since Nobel laureate Walter Kohn was recruited to direct the Institute for Theoretical Physics in 1979. Today, the National Research Council ranks UCSB’s doctoral program in physics as the fifth best nationwide.
The most valuable dividend of UCSB’s substantial contribution to the discovery of the Higgs boson, however, may be the most difficult to quantify. Discoveries like these turn the whole world’s eyes for one brief moment toward the mysteries of the universe, keeping alive that sense of wonder that hovers between inquisitiveness and understanding, driving us to explore even more. It’s what David Stuart likes to call “cosmic curiosity.” Explained the UCSB professor, “It’s the same reason that people look at the stars or fly to the moon.”