Model Organisms
Seeing Ourselves in Others

Researchers have long studied other organisms in hopes of better understanding the human body. The severe ethical issues raised by the prospect of using humans beings for biological studies, such as for studying the function of disease and the process of development, are self-evident enough, and this is why “model organisms” have been vigorously pursued as an alternative by researchers.
There are many qualifications such an organism must have: it must be similar enough to humans, in some way, that the knowledge gained is applicable to us; it usually needs to be simpler than us so that the process being studied is easier to figure out; it must be easy to grow in a laboratory setting, preferably in great numbers and relatively quickly. There are many organisms over the years that researchers have found to fit this description. These “models” have become the center of attention in many laboratories throughout the decades, and have brought us a much greater understanding of ourselves and how we are all related.
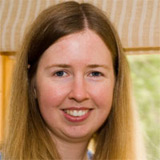
By the late 1800s, researchers realized the importance of using animal models, as many different factors began to fall into place. Until this time, many scientists had been trying to simply apply their knowledge of physical laws to understand physiology, but this was not working out very well. At the same time, with the advent of mass production, standardized equipment was becoming widely available to researchers. In 1900, attention was also brought back to the field of genetics, as the research done in the 1860s by Gregory Mendel, the “father of genetics,” was rediscovered. Researchers were soon looking for the best animals in which to study this novel field of genetics.
The Fly: While many would look upon the tiny fruit fly as a pest, scientists saw it as a great opportunity. The fruit flies (Drosophila melanogaster) have many attractive features: they are small, taking up little space in the laboratory and being relatively cheap to maintain; they reproduce quickly and in great numbers (a mature female can lay up to 100 eggs a day, and one fly goes through its lifecycle in about 10 days); and like humans, they are eukaryotes (which includes all animals, plants, fungi, and some single-celled organisms, with the defining characteristic being the presence of a nucleus), although fruit flies are much simpler than we are (with only 4 chromosome pairs to our 23 pairs).
During the first decade of the 1900s, a few research groups decided to try these new models out in their laboratories, and word of their appeal quickly spread; the fruit fly became the first widely accepted model organism in laboratories. Thomas Hunt Morgan at Columbia University was one of the first to get in on the fruit fly craze. He decided it would be a perfect model to study genetics, as there were a variety of obvious, physical mutations which different fruit flies had and passed on to their offspring, including different eye colors, body colors, and wing shapes, to name a few. These mutations could all be easily observed with a magnifying glass. He created his famous “Fly Room” to map these different physical mutations to separate locations on the fruit flies’ chromosomes. These efforts were spectacularly successful and consequently he helped make the fruit fly a prominent genetics model for researchers in the 1910s and 1920s, and was awarded a Nobel Prize in 1933 for showing that chromosomes carry genes, a concept central to our understanding of heredity.
However, as the tools available to researchers improved, so did their desire to investigate smaller and smaller components of the biological world around them. When the electron microscope became available to researchers in the 1930s, it drastically changed the focus of research; they could now magnify an image of a sample 500,000-fold. The previously obscured world of bacteria and viruses was no longer so hidden from view, though it was still far from understood.
The Bacteria: By the 1950s and 1960s, many groups were using the stomach bacteria Escherichia coli (E. coli) and bacteria viruses (“bacteriophages”) to understand genetics on a smaller, molecular basis. Rarer genetic mutations were studied using bacteria, as they can be quickly grown in huge numbers (E. coli doubles its population size about every 20 to 30 minutes under ideal conditions). E. coli can also be manipulated to take in foreign DNA and make more of it as if it were its own, or even turn the foreign DNA into protein; E. coli is now commonly used in laboratories to produce large quantities of DNA and protein that are from other organisms. For example, E. coli produces the vast majority of insulin used to treat diabetics in the world today.
Although bacteria are prokaryotes (while humans are eukaryotes), researchers also use bacteria to study cellular processes that are highly conserved (very similar) between prokaryotes and eukaryotes, such as gene regulation and proteins that play roles in disease. At the University of California at Santa Barbara (UCSB), Christopher Hayes’ laboratory investigates the function of ribosomes (the cellular machinery that creates proteins) in E. coli and David Low’s laboratory studies infectious E. coli.
The Mouse: While researchers learned a great deal from simple organisms like the fruit fly and E. coli, model organisms that are closer to humans were needed for many investigations. This is how the mouse came to the laboratory spotlight. Mice (Mus musculus) are very similar to humans not only genetically, but also physiologically; they can develop cancer and similar diseases as humans do, or be manipulated to develop diseases and carry genetic mutations. Additionally, mice have a relatively short lifespan and can reproduce in large numbers. Perhaps the researcher who is most responsible for the abundance of mice in laboratories today is Clarence Cook Little, who developed the first inbred mouse line in 1909 and went on to found The Jackson Laboratory in 1929 as a mouse cancer research facility. The Jackson Laboratory created many lines of mice for researchers to use globally, “normal” mice as well as mice that functioned as models of human diseases or cancers, ensuring that the mice used in laboratories around the world would be standardized. With the genome of the mouse sequenced in 2002, today these well-characterized mammals are often used in laboratory work as research transitions to the clinic, frequently in cancer and tumor research.
The Worm: One of the most widely used model organisms was a relatively late arrival to the scene. The appealing simplicity of the nematode (Caenorhabditis elegans, or simply C. elegans) prompted Sydney Brenner (Medical Research Council in Cambridge, UK) to use it and spread it to research laboratories in the 1960s. “The worm,” as it is fondly referred to by researchers, is an ideal model organism; among other appealing traits, it’s small (only 1 millimeter-long), can reproduce quickly and in great numbers (by self-fertilizing with hermaphrodites or introducing new genetics with rarer males), and is transparent, making it easy to see through under a microscope. Brenner was attracted to C. elegans because he wanted to study how the nervous system develops and thought that the essential processes would be similar between humans and the more “simple” multi-cellular worm. Brenner created a diagram of all of the neurological connections within the worm, and for his work received a Nobel Prize in 2002. The appeal of using C. elegans for studying development was soon shared by many other researchers, some who went on to map the destiny of every cell in the egg; all of the approximately 1000 cells that make up the adult worm can be traced to precursors in the egg. Today at UCSB, Joel Rothman’s laboratory continues to elucidate the intricacies of C. elegans’ development to better understand the process of development in general.
The Return of the Fly: Though the fruit fly (D. melanogaster) was heavily used in genetics research during the first couple decades of the 1900s, it was too complex a model to use for more detailed understanding of genetics at the time, and so it faded from the research spotlight for a while. In the 1970s it made a big comeback, as the field of developmental biology began to take off. The many, well-characterized mutant lines of fruit fly that had been used for genetic studies were now used in developmental studies; researchers could trace a specific, obvious mutation back to its developmental origin, and thereby learn about the cell lineages during development. Today at UCSB, Stephen Poole’s laboratory uses the fruit fly to study genetics during development, and William Rice’s laboratory uses it in evolutionary genetics studies. An even simpler eukaryote, the single-celled, freshwater ciliate Tetrahymena thermophila is used as a model for experimental cell and molecular biology at UCSB in the laboratory of Eduardo Orias, who recently celebrated 50 years of working at the university.
The Others: Several other model organisms are used in laboratories at UCSB, including a variety of plants (to study plant growth, fruit ripening, and host-microbe symbiosis, among other efforts), and animals that play key roles in ecological systems. The proximity of UCSB to the ocean has allowed it to develop extensive marine laboratories, perfect for the study of many types of unique marine organisms. This has allowed researchers to better understand some unusual stem cells, natural biomaterials, and fertilization and early development in the sea urchin (Strongylocentrotus purpuratus), which believe it or not is a very similar process to humans.
While not an “organism,” perhaps the most frequently used biology models today are cultured cells. (These are cells that were originally from an animal, but are grown separately, outside of the animal.) This allows researchers to study a wide variety of processes in human cells. Additionally, it bypasses many of the ethical concerns associated with using animals in studies. The field of stem cells, which Dennis Clegg at UCSB is heavily involved in, is perhaps at the forefront of using cultured human cells to better understand human processes such as development, cancer, and wound repair.
Using different models to answer different questions, scientists at UCSB and institutions around the world spend their days examining the delicate inner workings of life. The models may look different, but they all contribute a piece to the puzzle of understanding ourselves and our world.
For more on model organisms, see Angela N. H. Creager, Elizabeth Lunbeck, and M. Norton Wise’s “Science Without Laws: Model Systems, Cases, Exemplary Narratives,” Wikipedia’s article on “Model organisms,” Wikipedia’s article on History of Model Organisms, the website of “The Jackson Laboratory,” and the National Institutes of Health’s website on Model Organisms.
Biology Bytes author Teisha Rowland is a science writer, blogger at All Things Stem Cell, and graduate student in molecular, cellular, and developmental biology at UCSB, where she studies stem cells. Send any ideas for future columns to her at science@independent.com.