Genetically Modified World: Part II
Microorganisms with Amazing Abilities
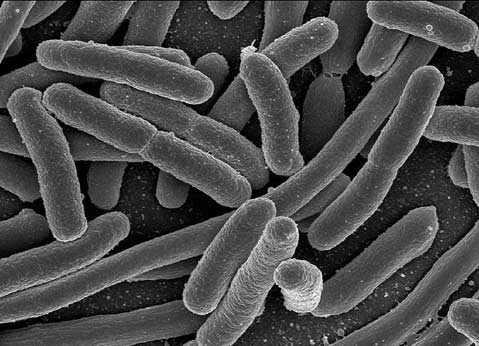
When thinking about genetically modified organisms (GMOs), the first things that usually come to mind are GM plants (which were discussed last week) and animals, which overlooks possibly the most important group of all: GM microorganisms.
Through decades of development, the products of these miniscule critters have come to affect our daily lives. They have already become essential to the medical and food processing industries. Perhaps one day, they will also be critical to the agricultural and energy-production businesses, among others.
The First GMO: In the early 1970s, Herbert W. Boyer and colleagues (at the University of California, San Francisco, and Stanford University School of Medicine) created what is generally considered to be the first genetically modified organism. That organism was a microorganism, specifically the bacteria Escherichia coli (E. coli). (While we’re most familiar with pathogenic E. coli strains through the headlines they occasionally make, there are actually many strains of E. coli that naturally live in our stomachs as a part of its rich, and essential, micro-flora.) Bacteria like E. coli have relatively simple genetics compared to plants and animals, which is part of why researchers have used them in genetic manipulation studies for decades. Additionally, E. coli reproduce quickly and can be grown in large numbers relatively cheaply.
So how did Boyer and colleagues “modify” the genetics of E. coli? Very simply put, the researchers put the DNA of a gene known to confer antibiotic resistance into the bacteria, and showed that the modified bacteria became resistant to the antibiotic. But, of course, it’s a bit more complicated than this. The antibiotic resistance gene was not sent into the bacteria all alone; it was delivered in a package called a “plasmid,” which is a piece of DNA (usually circular) that tells the cell to make more copies of the plasmid—along with any genes that are in it, like the antibiotic resistance gene. Boyer’s team showed that two different plasmids could be cut open and recombined to make a new, unique plasmid. (This is why it is called “recombinant” technology.)
This laid the foundation for a whole new enterprise.
What exactly were the implications of Boyer’s work? That desired proteins could be made by bacteria in vast quantities, without the need for the harsh chemicals and extreme conditions that chemical synthesis often requires. On a single plasmid, researchers could not only put a gene that makes the bacteria become antibiotic resistant, but they could also insert a gene that would make the bacteria manufacture some protein of interest. While bacteria are pretty good at incorporating foreign DNA they’re exposed to, compared to most organisms, such efforts are still nowhere near 100 percent successful. Consequently, to make sure the bacteria have taken up the plasmid researchers want them to take up, they can expose the bacteria to an antibiotic. The ones with the plasmid will live, the others will die. With the desired bacteria selected out, they can be easily, rapidly grown to great numbers and then stimulated (by exposure to a certain protein) to make the desired protein. Because the bacteria have been made to focus all their energies on producing this protein, they often will die as the protein is being harvested, but produce a lot of protein in the process. This strategy has become an essential technique, used routinely in laboratories across the world today for a variety of purposes.
Medical Applications: Many quickly realized the implications of this new recombinant technology. In the mid-1970s, Boyer went on to found Genentech, often considered the first biotechnology company. In 1977, the first human protein created in another organism was produced; Genentech made E. coli that could turn out the human protein somatostatin (which regulates hormones in the body, among other functions).
A year later, Genentech succeeded in modifying bacteria to make human insulin, which became the first GMO-produced product on the market in 1982. Insulin is needed to treat patients with type 1 diabetes, and can control the progression of type 2 diabetes in some patients. Insulin specifically stimulates the body to take sugar (specifically glucose) out of the blood stream and put it into different tissues (i.e. the liver, fat tissue, and muscle), where it is stored until needed. When insulin levels are low, or when a person becomes insulin resistant (their tissues no longer respond to normal levels of insulin), then high blood sugar levels can result, which can cause diabetes. Before Genentech created a “recombinant” source of human insulin, all medical insulin was harvested from the pancreases of pigs or cows. In 2007, sales of insulin products estimated around $8 billion globally, and they will almost surely increase with time. (All GM-produced proteins must be chemically pure and safe, by the way, just as any non-GM protein must, to be approved for use.)
In the 1980s, Genentech competed with other biotechnology companies to be first in creating recombinant blood-clotting factors. Why clotting factors? Clotting factors are necessary for people with hemophilia to survive. But in the early 1980s, significant amounts of stored clotting factors that had been derived from human plasma were found to be contaminated with HIV. Genentech won the race; its scientists were able to make several clotting factors in the laboratory (e.g. anti-hemophilic factor, or factor VII, and factor IX).
By now, a wide variety of drugs, hormones, and other products have been created in microorganisms for the medical market. This includes erythropoietin (which regulates red blood cell production), interferons (which stimulate the immune system), vaccines (e.g. against the Hepatitis B virus), human growth hormone (hGH), and many more. Most recently in the medical field, bacteria are in clinical trials that may prevent cavities, and other bacteria are being created that may possibly block HIV infection.
It’s What’s for Breakfast! Today, many of the food additives we consume on a daily basis are made by GM microorganisms. To name just a few, the list includes vitamins (B2, C), amino acids that improve flavors (e.g. aspartame), food additives (e.g. xanthan), food preservatives (e.g. nisin), enzymes used in food during specific chemical reactions (e.g. making cheese, breads, certain alcohols, and some sugars), and many more. While some of the microorganisms used may naturally make these products and are only coaxed into producing higher quantities, other microorganisms do not and must have the foreign gene inserted in them to do so.
Release into the Environment: The U.S. Environmental Protection Agency (EPA) approved the first use of genetically modified bacteria in the environment in 1985. In that particular case, researchers had taken bacteria that normally encourage ice formation on plants (specifically Pseudonomas syringae and P. fluorescens) and got rid of a gene that the bacteria needed to do this. Consequently, plants colonized with these modified bacteria do not form frost until around 23 degrees Fahrenheit, saving them from damages brought upon plants by an early frost, or unusually cold weather.
Researchers soon explored another agricultural area that could greatly benefit from GM bacteria: the creation of improved nitrogen-fixing bacteria to help increase legume growth. Legumes (which include beans, lentils, peanuts, soy, alfalfa, clover, and more) are distinguished by their ability to capture nitrogen from the atmosphere, which they accomplish through a symbiotic relationship with a group of bacteria (rhizobia) that live in their roots. The bacteria Sinorhizobium meliloti, which can colonize multiple types of legumes, has been of particular interest. In some field studies, legumes with genetically modified S. meliloti had improved plant growth.
However, some of these studies have revealed an area of concern for using GM microorganisms: While the introduced bacteria (which is dependent on the legume hosts) was often undetectable after a few weeks or a year, sometimes it persisted in soil for more than two years. There have also been concerns that the genes of persistent GM microorganisms may be transferred to other, native bacteria, or to the organisms that consume the bacteria. Ongoing work is being done to address such issues.
More recently, genetically modified bacteria has been used to clean up the environment. In one such example, researchers have used rhizobia bacteria (specifically our friend S. meliloti again) and modified them to be able to break down a compound related to TNT. When alfalfa seeds were coated with these modified bacteria and planted in soil with the compound, the bacteria could reduce the pollution levels while stimulating plant growth.
In the works …: A variety of creative applications for using genetically modified microorganisms have been explored over the last decade.
In 2005, light-sensitive E. coli were created and used to create a kind of slow-action, high-resolution camera.
An array of yeast strains have been modified that can convert sugars to ethanol (which we currently mostly harvest from corn), which could be a valuable alternative energy source. Similarly, many efforts have been invested in creating gasoline (crude oil) from microorganisms.
Just last week, there was a breakthrough in generating stronger, lighter silk from bacteria. They’ve become more efficient than ever (generating one to two grams of silk per liter of bacteria), but how to efficiently put these silk proteins together into useable threads remains a big problem.
The Future: GM microorganisms have certainly been a boon for the medical and food industries, not to mention general laboratories everywhere. They also hold promise for creative applications and possibly for well-needed alternative energy sources. However, there is still concern over the release of GM microorganisms into the environment; in general, more observational studies on the effects of these microorganisms on their new habitats would be beneficial.
Check “Biology Bytes” next week for Part III of this series on the “Genetically Modified World,” where we will explore the amazing array of genetically modified animals that have been created.
For more on genetically modified crops see Dominic W. S. Wong’s “The ABCs of Gene Cloning,” the National Research Council’s workshop summary on “Genetically Engineered Organisms, Wildlife, and Habitat,” Wikipedia’s article on “Genetically Modified Crops,” Gary Walsh’s article on “Therapeutic insulins and their large-scale manufacture,” Steven W. Pipe’s article on “Recombinant Clotting Factors,” EPA’s approval of “First use in Environment of Genetically Altered Bacteria,” Mareike Viebahn et al.’s article on the “Effect of Genetically Modified Bacteria on Ecosystems and Their Potential Benefits for Bioremediation and Biocontrol of Plant Diseases,” the use of genetically modified bacteria to make pictures, or using genetically modified bacteria to make stronger silk.
Biology Bytes author Teisha Rowland is a science writer, blogger at All Things Stem Cell, and graduate student in molecular, cellular, and developmental biology at UCSB, where she studies stem cells. Send any ideas for future columns to her at science@independent.com.