Direct Reprogramming
Changing a cell’s identity
The idea that a small piece of skin could be taken from a person and turned into a kidney useful for a transplant sounds like science fiction. But in the last few decades such amazing progress has been made in the stem cell field as to suggest that someday in the not-so-far future, this may be reality.
Stem cells hold great potential for treating diseases and regenerating tissues. One key reason for this is that stem cells can turn into many different types of cells. Equally valuable is their ability to be patient-specific; cells can be taken from a patient and used to treat that same patient, bypassing potential immune rejection. Possibly embodying these two traits more than any other stem cells, human induced pluripotent stem cells (iPSCs), first created in 2007, can become any cell type in the body and made from a patient’s own cells. (For more on iPSCs, see “Adult Cells Turned Stem Cell.”)
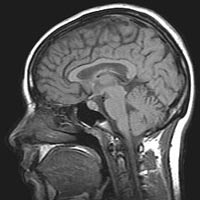
Although iPSCs are not yet being used clinically, the theoretical procedure for making iPSCs for treatments is time-consuming. Adult cells must first be obtained, then “reprogrammed” into an embryonic-like state where they can potentially turn into any cell type, and lastly coaxed into becoming the final desired cell type. “Reprogramming” involves forcing the adult cells to produce factors that are normally only made in embryonic cells. These factors (called transcription factors) change the proteins the adult cell produces so that it starts to make embryonic proteins, eventually making it look and function like an embryonic stem cell. Just as embryonic stem cells can become any cell type, so can their mimics, the induced pluripotent stem cells.
Researchers over the last few decades have been investigating ways to bypass the intermediate embryonic-like state and reprogram adult cells directly into the final desired cell type, an approach called “direct reprogramming.” Their work has shown that this is clearly possible, although the underlying mechanisms remain poorly characterized. Most of these studies have been done using a few cell types: muscle, blood, pancreatic cells, and neurons.
In the late 1980s, researchers found that many different types of cells could be turned into muscle cells, in the first direct-reprogramming experiments done with transcription factors. In 1987, Dr. Harold M. Weintraub and colleagues at the Department of Genetics Fred Hutchinson Cancer Research Center in Seattle, Washington, found that when fibroblasts (cells in connective tissue that are important for wound healing) were forced to produce a transcription factor the researchers named MyoD, the fibroblasts became muscle cells. MyoD was later found to be important in turning cells into muscle during normal development.
Because it worked so well in fibroblasts, MyoD was tested for its ability to reprogram other cell types into muscle. Indeed, in the early 1990s, other groups found that MyoD could in fact make skin cells, cells from cartilage, and cells from the retina, among others, all become muscle. However, not all cells were easily persuaded to change their fates; liver cells couldn’t become muscle. Unlike many scientists who have made ground-breaking discoveries, Dr. Weintraub lived to see his discovered transcription factor, MyoD, recognized as an important factor before he died of cancer in 1995, at the age of 49.
The 1990s saw the arrival of many more important transcription factors to the direct reprogramming scene. One biological system that had its reprogramming abilities heavily explored was blood. A key group of stem cells called hematopoietic stem cells (HSCs) is responsible for creating all of the different blood cells the body needs to combat infections and deliver oxygen to its organs, among other essential duties. The creation process is called hematopoiesis (which literally means “to make blood”). During hematopoiesis, an HSC turns into one of two major blood groups. One group contains white blood cells, red blood cells, and platelets. The second group is made up of lymphocytes. (For more on hematopoietic stem cells, see “Stem Cells That Treat Leukemia, Lymphoma, and HIV.”)
In 1995, Dr. Thomas Graf’s group at the European Molecular Biology Laboratory in Heidelberg, Germany, found that changing the amount of a factor called GATA-1, present in white blood cells (monocytes), could make them turn into other kinds of blood cells from the same group: other white blood cells (eosinophils), red blood cells (erythrocytes), or platelets (thrombocytes), which are essential for blood clotting. This correlated with the normal role of GATA-1 during hematopoiesis; different amounts of GATA-1 are present in these different blood cell types, and the presence or absence of GATA-1 controls what fate these cells usually have. While this finding might not seem as impressive as being able to turn many different types of cells into muscle, these experiments were done in the well-characterized blood system, so that even small cell-fate changes could be better understood.
Over the last decade, Dr. Graf’s group has continued to better understand reprogramming in the blood system. In 2004, his researchers found that when lymphocytes were forced to produce key transcription factors (a group called C/EBPs), the lymphocytes could become white blood cells—making one blood cell become a blood cell type from the other major blood cell group. This makes sense with C/EBPs normal role in hematopoiesis; they are necessary for white blood cells to develop.
While it seemed easy enough to make cells become muscle, or make blood cells become other kinds of blood cells, it took a great amount of effort to make such direct reprogramming possible in other parts of the body. It was found to be possible, and in 2008 Dr. Douglas A. Melton’s group at the Harvard Stem Cell Institute, at Harvard University, in Cambridge, Massachusetts, reported that they had made one kind of cell in the pancreas (specifically, exocrine cells) become another, functionally different type of pancreatic cell (beta-cells). While exocrine cells secrete digestive enzymes used by the intestine, beta-cells are important for producing insulin. Since diabetes can be caused by a lack of insulin, it is very appealing to be able to create more insulin-producing cells.
Dr. Melton’s group screened over 1,000 transcription factors to find which could make the exocrine cells turn into beta-cells. Based on how the exocrine cells responded to the factors, the group narrowed down their list to a few key factors; eventually three used together made them into cells like beta-cells. Not only did they look like beta-cells, but they also produced proteins made by beta-cells, and generated insulin. This discovery renewed reprogramming efforts in other cell types, and gave new hope to diabetes research.
However, with the exception of MyoD and muscle, it was still unclear whether a cell could be reprogrammed into a very distantly related cell type. This brings us to the most recent direct reprogramming breakthrough. Last month, Dr. Marius Wernig’s group at the Stanford University School of Medicine showed that fibroblasts from the tails of mice could be turned into nerve cells, or neurons. After screening 19 candidate factors, the researchers found three (all of them normally involved in neuronal development) that turned the fibroblasts into functional neurons.
While it is clearly possible to directly reprogram one cell type into another, it is still very unclear how this happens. For instance, it is not known whether a “directly reprogrammed” cell actually directly becomes the final cell type seen, or whether it undergoes a transition state before settling on the final cell type. Aside from using transcription factors, several other methods of reprogramming are being pursued (such as using microRNAs, using chemicals, or altering chromatin states). It may turn out that the most effective approach is to combine these different methods.
While direct reprogramming results are quite promising, they are still at a very early stage; most of the studies reported have been in mice or mouse cells and must be repeated using human cells before they can be considered for use in humans. However, if such reprogramming studies continue to be successful, they may play a key role in creating patient-specific cells for treatments and regenerative medicine, as well as ideal cells for studying development and diseases that occur when cells lose their identity, such as cancer.
For more on direct reprogramming, see Teisha Rowland’s “All Things Stem Cell post on Direct Reprogramming: Turning One Cell Directly Into Another,” The National Institute of Health’s Stem Cell FAQs, or, for a visual explanation of terms used, see All Things Stem Cell’s Visual Stem Cell Glossary.
Biology Bytes author Teisha Rowland is a science writer, blogger at All Things Stem Cell, and graduate student in molecular, cellular, and developmental biology at UCSB, where she studies stem cells. Send any ideas for future columns to her at science@independent.com.